Science Spotlight: Gravitational Waves
Gravitational waves were discovered in September, proving Einstein’s theory from 100 years ago.
A chirp. That’s how Senior Editor and Planetary Evangelist Emily Lakdawalla described the recordings captured by LIGO on September 14, 2015 of gravitational waves from two colliding black holes. The pair of black holes, with masses of 36 and 29 solar masses – 332,946 earth masses is equal to one solar mass – collided 1.3 billion years ago, producing 3.6 x 1049 watts of power. That’s more power than all light radiated from all stars in the observable universe.
Physics teacher Karen Phillips has followed LIGO for 30 years now. Her daughter, Emily Phillips Longley ‘12 is a senior at Carleton College and works with Dr. Nelson Christensen and other LIGO physicists both at Carleton and at the University of Glasgow in Scotland. When describing what gravitational waves are, Phillips compares them to photons and energy: “we have an electric field and as a consequence of energy and magnetism being connected, you get electromagnetic waves in the form of a photon. We have a gravitational field, but how is that energy transferred in that field? Through gravitational waves.”
This connection between the gravitational field and energy is supported by physics teacher Steve Kaback, explaining “a gravitational wave is a disturbance in the gravitational field that is created when you have a mass undergoing an acceleration,” where the masses scientists analyzed in September were the two black holes accelerating towards each other. The gravitational field, as Kaback explains, is “a representation that had to do with the curvature of the space-time continuum. Warping the space time continuum [is analogous to] having one axis is time, the other however axis is all three dimensions.”
Complicated as this may sound, a simpler way to think about gravitational waves is through thinking of oscillations in three dimensions. If time is continuous, then masses in three dimensional space will oscillate in a way that minutely changes the distances in space. Yet, as Phillips explains, because the movement of the detectors’ mirrors from the gravitational wave is around 10-21 meters – about one trillionth the diameter of an atom, the changes in the space-time continuum can only be observed with the use of extremely specific equipment such as an Interferometer.
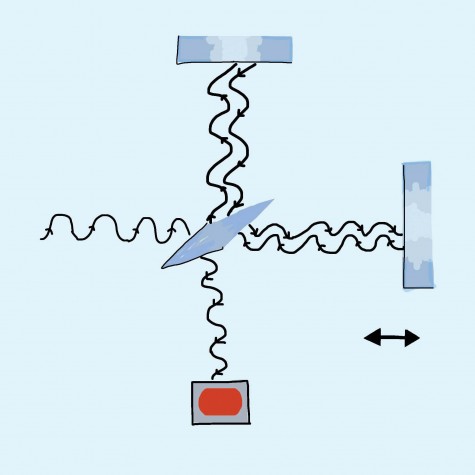
The Interferometer was used to detect the gravitational waves.
LIGO (Laser Interferometer Gravitational-Wave Observatory) is one of three gravitational-wave Interferometers in the world. LIGO has two facilities, one in Louisiana and one in Washington State–3,002 kilometers apart. Yet, despite this seemingly large distance between the two facilities, since gravitational waves travel close to the speed of light, the difference in arrival time of the gravitational waves is roughly 10 milliseconds (.01 seconds).
The way Interferometers detect gravitational waves is through by using lasers. A laser is emitted from a source, which is then split by a mirror into two arms. The arms are perpendicular to each other and are exactly four kilometers in length. Because gravitational waves distort the space-time continuum, the distances that the light travels are altered very slightly, thus the time for the light to travel down one arm is slightly longer than the time for the other arm. When the two light beams reach the detector, they are slightly out of phase with each other, creating destructive interference with a period that produces a signal.
Basically, the Interferometers need to be extremely accurate. Kaback explains that this was once explained to him using the analogy of “trying to find a variation the diameter of a nickel on the surface of Saturn.”
Gravitational waves may be difficult to understand, but the implications for technology, may solve problems that we don’t even know exist.
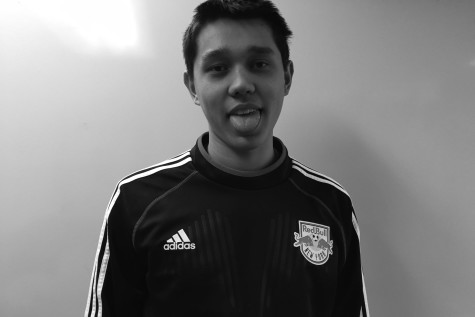
Deniz has been an "editor" since the beginning of his junior year and declares himself the "bananagram king." He enjoys the color brown and long walks...